INTRODUCTION
MATERIALS AND METHODS
Plant material and field cultivation
Application of plant growth retardants
Extraction and analysis of fatty acids
Extraction and analysis of lignans (SDG, secoisolariciresinol diglucoside)
Extraction and identification of endogenous GAs
Quantification of endogenous GAs
Statistical analysis
RESULTS AND DISCUSSION
INTRODUCTIO
Plant growth retardants are applied in agronomic and horticultural crops to reduce unwanted longitudinal shoot growth without lowering plant productivity (Hedden & Kamiya, 1997). Most growth retardants act by inhibiting gibberellin (GA) biosynthesis. To date, four different types of such inhibitors are known: Onium compounds, such as chlormequat chloride, mepiquat chloride, chlorphonium, and AMO-1618, which block the cyclases copalyl-diphosphate synthase and ent-kaurene synthase involved in the early steps of GA metabolism (Rademacher, 2000). Flax is used for fiber and oil is an annual crop belonging to the family Linaceae. Flaxseed meal is 35 to 40% proteins, and together with cottonseed and sunflower supplies about 23% of the world’s oilcake and meal. In flax culture, it has been tried to raise seed and oil productivity. Mepiquat chloride and chloromequat chloride were found to restrict vegetative growth, thus enhancing reproductive organs. Mepiquat chloride as one of gibberellin biosynthetic inhibitors has been found to restrict the vegetative growth in the cost of enhanced reproductive organs. It was reported that mepiquat chloride improved photosynthetic efficiency and application of mepiquat chloride to cotton plants increased protein and oil yields and also caused a general decrease in the oil saturated fatty acids, associated with an increase in unsaturated fatty acids (Osman, 1985).
Mepiquat chloride and trinexapac-ethyl tend to be shorter and more compact than untreated plants (Hodges et al., 1991; Jung et al., 1975; Kerby, 1985; Reddy et al., 1992; Stuart et al., 1984; Willard et al., 1976). Mepiquat chloride, chloromequat chloride, and daminozide also have been associated with increased photosynthesis through increased total chlorophyll concentration in plant leaves (Nepomuceno et al., 1997; Wu et al., 1985). Sawan et al. (1991) indicated that application of mepiquat chloride to cotton plants increased protein and oil yields and also caused a general decrease in the oil saturated fatty acids, associated with an increase in unsaturated fatty acids. Al-Gharbi & Yousif (1989) observed that application of chloromequat chloride increased sunflower oil seed content. In a previous our study, it revealed that mepiquat chloride increases seed oil content and promotes unsaturated fatty acids in flax under open field condition (Kim et al., 2011).
Accordingly, the current study aims to compare the cultural difference between open field (data not shown and refer to previous publication literature, Kim et al., 2014) and greenhouse on change of oil content, and seed yield as affected by two plant growth retardants in flax plants.
MATERIALS AND METHODS
Plant material and field cultivation
Experiment was conducted at the experimental field of Gyeongsangbuk-do Provincial Agricultural Research & Extension Services, Daegu, Korea. Flax (Lusitatissimum L. cv. Hwanamama) seeds were planted in 4.8 m (16 rows) with 6.0 m (row length) plots covered with black polyethylene vinyl under greenhouse on March 15 in 2017. Prior to seeding, fertilizer was supplied with nitrogen, phosphorus, and potassium at the 10, 2.5, and 9kg/10a, incorporating as basal and top dressing (7:3 ratio, w/w) to the soil.
Application of plant growth retardants
Two growth retardants, paclobutrazol (PB) and prohexadione- calcium (PC) were treated to flax plants. The concentrations of each growth retardant were as followed: PB 40, 80, 160 ppm and PC 500, 1000, 2000 ppm, respectively. Each plant growth retardant was foliar-sprayed at 55 days after seeding. A randomized complete block design with four replications was used. Flax seeds were harvested on 17 August in 2017. Oil content and fatty acid composition were determined from matured flax seed.
Extraction and analysis of fatty acids
Flax seed (5 g fresh weight) was extracted by percolating with diethyl ether 100 ml using soxhlet apparatus. For analysis of fatty acid, the extracted solvent derived from soxhlet was evaporated and concentrated. Fatty acid methyl esters were prepared from in 200 ml of a 1% (w/v) solution of sodium methoxide in methanol as described previously (Hitz et al., 1994). After 20 min of incubation at room temperature, fatty acid methyl esters were recovered by the addition of 250 ml of 1 M sodium chloride and extraction with 250 ml of heptane and analyzed using a gas chromatogram (HP 5890 Series II, Wilmington, DE). Fatty acid methyl esters were resolved using an Omegawax 320 column (30 m×0.32 mm ID, 0.25 µm, CN 24152, Supelco, PA, USA), and the oven (HP 5890 Series II, Wilmington, DE) temperature was programmed from 185°C to 215°C at a rate of 2.5°C min-1.
Extraction and analysis of lignans (SDG, secoisolariciresinol diglucoside)
Extraction and analysis of SDG (secoisolariciresinol diglucoside) followed the reference (Meagher et al., 1999). Defatted flax powder was extracted with 50 ml of 80% methanol for 5 h at 55°C in a shaking water bath. The methanolic extract was filtered and concentrated by rotary evaporation. The resulting aqueous extract was hydrolyzed with 0.8 ml of 1M hydrochloric acid for 1 h at 100°C. The acid hydrolysate was diluted with water and extracted twice ethyl acetate/ hexanes (1:1). The dry samples were redissolved in methanol, filtered and applied to the HPLC (Series 1100, Wilmington, DE). HPLC semipreparative separations were carried out on a Beckman 114M HPLC system with a Microsorb semipreparative C18 column. The HPLC system was equipped with an HP 1100 DAD, with detection set at 280 nm and 400 nm for identification. Elution was carried out with a flow rate of 0.6 ml/min using following solvent systems: solvent A, water/glacial acetic acid (99.8:0.2 v/v) and solvent B, acetonitrile. An initial ratio of 70A:30B was followed by a linear gradient to 50A:50B, over 55 min, then back to 70A:30B, for equilibration of the system over 5 min.
Extraction and identification of endogenous GAs
Extraction and analysis of gibberellin metabolites followed the reference (Lee et al., 1998). The seeds harvested were immediately frozen in liquid nitrogen and stored at -80°C. When all the required materials for GA analysis had been collected, the samples were lyophilized for 48 h. The extraction of endogenous gibberellins was followed as described by Lee et al. (1998). The GAs were chromatographed on a 3.9×300 mm μBonda-Pak C18 column (Waters, MA, USA) and eluted at 1.5 ml min-1 with following gradient: 0 to 5 min, isocratic 28% MeOH in 1% aqueous acetic acid; 5 to 35 min, linear gradient from 28 to 86% MeOH; 35 to 36 min, 86 to 100% MeOH; 36 to 40 min, isocratic 100% MeOH. Up to 50 fractions of 1.5 ml each were collected. Small aliquots (15㎕) from each fraction were taken, and radioactivity was measured with liquid scintillation spectrometry (Beckman coulter LS 1801, CA, USA) to determine accurate retention times of each GA based upon the elution of 3H-GA standards. The fractions were dried on a Savant Speedvac and combined according to the retention times of 3H-GA standards and previously determined retention times of the labeled (deuterated) GA standards. GAs were quantified using [17, 17-2H2]-GAs (20 ng each) as internal standards (purchased from Prof. L. N. Mander, Australian National University, Canberra, Australia).
Quantification of endogenous GAs
The three prominent ions were analyzed by GC-MS-SIM (6890N Network GC System and 5973 Network Mass Selective Detector, Agilent Technologies, CA, USA) with dwell times of 100 ms. Endogenous GA contents were calculated from the peak area ratios respectively and retention time was determined by the hydrocarbon standards to calculate the KRI value. All results are presented as mean±standard deviation(SD).
Statistical analysis
Two-way ANOVA of mean values was performed to determine the significant level (p ≤ 0.05 or 0.01) among the treatments in a comparison with control. In addition, Duncan’s Multiple Range Test was also performed for the different treatments using Statistical Analysis Software (SAS, version 9.1, NC, USA).
RESULTS AND DISCUSSION
In our previous study, plant growth retardants (chloromequat chloride, paclobutrazol, and prohexadione-Ca) have shown that higher concentration of foliar application affected the change of growth, oil content, fatty acid composition when flax plants were grown in open field condition (Kim et al., 2014). Thus, the study was conducted to determine the change of oil content, composition of fatty acids, seed yield as affected by two plant growth retardants under greenhouse compared to that of open field condition. During growing season from sprouting to harvesting periods, air mean temperature in greenhouse was always 4.7 to 10.3°C higher than that of open field (data not shown) and the highest difference between greenhouse and open field in air mean temperature was recorded by 10.3°C during full ripening periods (from 15 June to 10 August). Plant height of flax plants following foliar application with paclobutrazol (40, 80, and 160 ppm) and prohexadione-Ca (500, 1000, and 2000 ppm) was reduced when it applied at 55 days after seeding compared to the control (Table 1). There was also significant difference between three paclobutrazol treatments and three prohexadione-Ca treatments. Kim et al. (2014) reported that the paclobutrazol and prohexadione-Ca treatments with foliar application significantly reduced the plant height when it grown in open field. In comparison with this study, plant height was much more reduced in the plant grown under greenhouse than that of open field conditions. The results suggested that high ambient temperature might be caused synergetic effect of paclobutrazol and prohexadione- Ca under greenhouse compared with result of open fields. The number of branch per plant treated with prohexadione-Ca differed significantly from that on the Control. The results clearly indicated that prohexadione-Ca treatment caused more branched in higher concentration than that of lower concentration compared to the Control. Increased ripening seed rate was observed in foliar application of two plant growth retardants, furthermore flax seeds showed more ripened seed rate in prohexadione-Ca treatment than that of paclobutrazol treatment. The highest ripening seed rate was recorded to 94.5% in 2000 ppm prohexadione-Ca, resulting 10.3% increment compared with the control. Foliar application of paclobutrazol and prohexadione-Ca affected the increasing thousand seed weight compared with the Control although thousand seed weight remained almost unchanged between the applications of the two plant growth retardants. Compared with the control, the different spaying concentration of paclobutrazol and prohexadione-Ca had few impact on reduced plant height, the increased branch, capsule, and higher ripening seed rate with elevated thousand seed rate compared with the control. Those promotive effects have contributed to a significant increase in seed yield compared to the control under greenhouse. Application of paclobutrazol and prohexadione-Ca caused markedly increases in flax seed production accompanying by positively promoted growth characters such as number of capsule, ripened seed rate and thousand seed weight. Foliar application with two plant growth retardants had the increased seed productivity by higher concentrations and it was more effective in prohexadione-Ca than in paclobutrazol. In comparing to our previous results (Kim et al., 2014), application of two plant retardants caused the flax at open field to have higher seed production than flax grown under greenhouse.
Table 1. Effects of two plant growth retardants on the growth characteristics and seed yield in flax grown under greenhouse condition.
Different letters in each column indicate significant difference at p < 0.05 by Duncan’s multiple range test.
Change of oil content and fatty acids composition in flax seed treated with two plant growth retardants are presented in Table 2. In two plant growth retardants, the highest oil content was observed in flax treated with PC, whereas the lowest oil content was showed in flax treated with PB. We had reported that the foliar application with mepiquat chloride causes higher oil content than trinexapac-ethyl. Furthermore, it was also revealed that the treatment of mepiquat chloride causes increased oil content when the higher concentration of mepiquat chloride was applied. Among change of fatty acid composition, saturated fatty acids such as palmitic acid and stearic acid showed relative lower content compared to the unsaturated fatty acids like oleic acid, linoleic acid, and linolenic acids under greenhouse.
Table 2. Changes in oil content and fatty acid composition in flax seedlings by the application of two plant growth retardants under greenhouse condition.
Different letters in each column indicate significant difference at p < 0.01 by Duncan’s multiple range test.
Oil content and ratio of unsaturated fatty acids were higher in flax seeds grown in greenhouse than that of open field conditions. Two plant growth retardants have well known to inhibit the biosynthesis of gibberellins in higher plants. Decreased endogenous gibberellins affect favorable growth characteristics such as reduced plant height, compacted stem internodes, and it was applied in agronomic and horticultural crops to reduce unwanted longitudinal shoot growth without lowering plant productivity (Matysiak, 2006). Enzymes, similar to the ones involved in GA biosynthesis, are also of importance in the formation of abscisic acid, ethylene, sterols, flavonoids, and other plant constituents (Lubna et al., 2018). Changes in the levels of these compounds found after treatment with growth retardants can mostly be explained by side activities on such enzymes (Jaleel et al., 2010).
The bioactive gibberellin content (GA1 and GA4) was determined by GC-MS from flax seeds treated with PB and PC (Table 3). In our previous study, it was first reported that eight gibberellins were identified and quantified operating two different gibberellin biosynthetic pathways, early C-13 hydroxylation and non C-13 hydroxylation routes. Two endogenous bioactive gibberellins (GA1 and GA4) in flax seeds were commonly decreased by PB and PC.
Table 3. Changes in endogenous gibberellin content of flax seedlings by the application of plant growth retardants under greenhouse.
Different letters in the column indicate significant difference at p < 0.01 by Duncan<rsquo;s multiple range test.
Flaxseed has known to be rich source of lignans such as secoisolariciresinol and matairesinol (Peterson et al., 2010). In the present study, it was the first report of the lignans being determined in seeds of flax plants treated with plant growth retardants grown in greenhouse.
Fig. 1 shows SDG (secoisolariciresinol diglucoside) content of whole flaxseed as affected by different concentrations of two plant growth retardants. The results showed that the flax plants applied with PB and PC produced higher SDG content with increased concentrations. In particular, PC treatment with 1000 and 2000 ppm showed significantly much higher SDG content compared with the control and PB treatments.
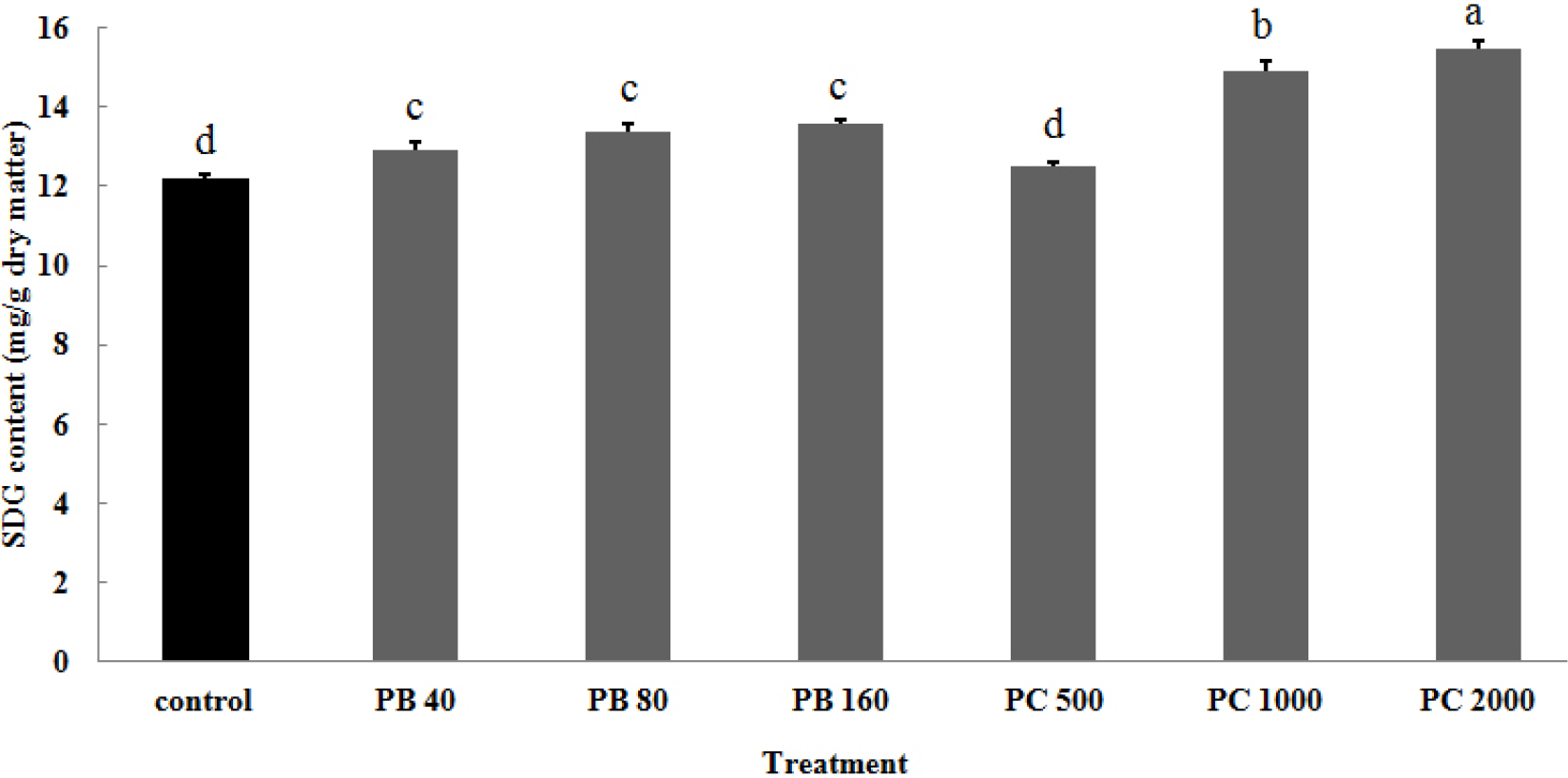
Fig. 1.
Change in SDG content of whole flax seedlings by the application of two plant growth retardants under greenhouse condition. PB, paclobutrazol; PC, prohexadione-ca. Vertical bars represent mean ± SD (n = 3).
Different letters in each bar indicate significant difference at p < 0.01 by Duncan’s multiple range test.
The SDG content from defatted flaxseed was also determined (Fig. 2). The SDG content of defatted flaxseeds treated with two plant growth retardants were 22.1 to 26.7 mg per dry weight. The higher concentration applied to flax, the more SDG content increased. The more significant increase of SDG content was observed in much higher concentration of PC treatment.
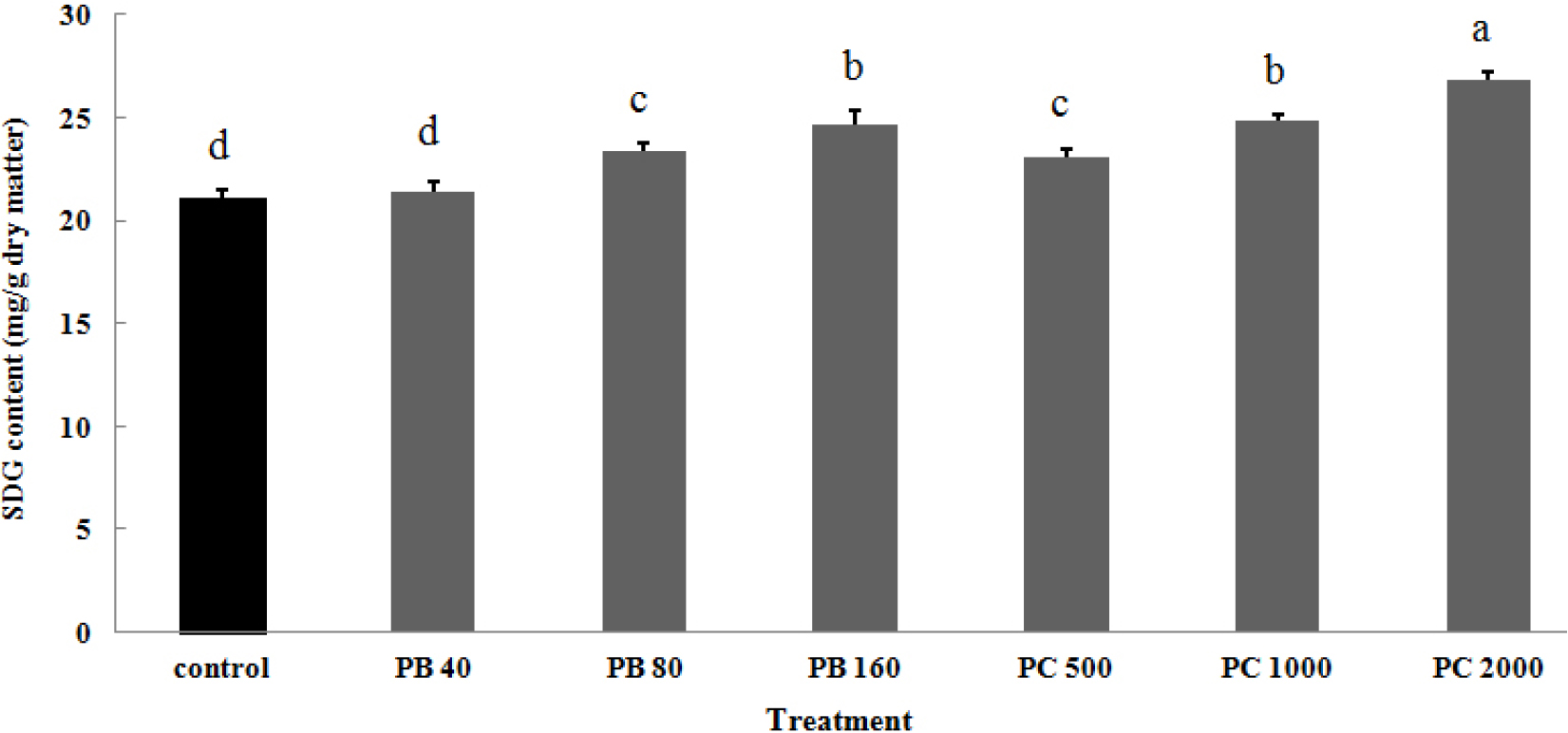
Fig. 2.
Change in SDG content of defatted flax seedlings by the application of two plant growth retardants under greenhouse condition. PB, paclobutrazol; PC, prohexadione-ca. Vertical bars represent mean ± SD (n = 3).
Different letters in each bar indicate significant difference at p < 0.01 by Duncan’s multiple range test.
Taken together, the results suggest that higher concentration of plant growth retardant (PC) increased flaxseed yield and oil content increasing linolenic acid content. The optimal concentration of PC treatment was observed in 2000 ppm. It concludes that the foliar application of 2000 ppm PC may be useful for the increasing oil and higher seed production in flax plants.