서 론
식물형질전환(Plant transformation)
아그로박테리움 밀 형질전환(Agrobacterium-mediated wheat transformations)
유전자총(Biolistic wheat transformation)
밀 형질전환을 이용한 밀 생명공학 연구현황
적 요
서 론
밀은 벼, 옥수수와 더불어 세계 3대 식량작물이다. 세계 생산 면적의 약 17%를 차지하며 세계 인구의 40% 이상이 식량으로 사용되고, 전 세계 인구가 소비하는 영양 칼로리와 단백질의 20%를 차지하는 등 여러 방면에서 중요한 식량작물이다(Gupta et al., 2008). 국내에서도 연간 32.1 kg 소비되는 주요 식량작물이지만, 국내 밀 생산량은 년간 3만톤이 안되는 수준으로 밀 자급률이 1%도 되지 않아 거의 전량을 수입에 의존하고 있다(MAFRA, 2019).
이렇듯 밀은 국내외에서 중요한 식량작물이지만 식물병, 비생물학적 스트레스(Abiotic stress), 밀 관련 질환 등 극복해야 할 여러가지 문제점이 있다. 먼저, 주요 밀 식물병인 녹병, 붉은 곰팡이병, 흰가루병 등이 세계적으로 많은 피해를 입히고 있다. 밀 녹병(rust)은 밀 잎녹병(leaf rust), 줄기녹병(stem rust), 줄녹병(strip rust) 세가지 종류가 있으며 각 주요 원인균으로 잎녹병균은 Puccinia recondita, 줄기녹병균은 Puccinia graminis, 줄녹병균은 Puccinia striiformis Westend. f. sp. tritici이다(Cummins & Hiratsuka, 2003; Duplessis et al., 2011; Figueroa et al., 2018). 녹병은 잎과 줄기에 주황색 또는 갈색 작은 반점이 점점 퍼지며, 감염된 부위는 갈색, 검은색으로 변한다(Cummins & Hiratsuka, 2003). 서늘하고 습한 온대지역에서 흔히 발생하고 발병지역에서 평균 밀수확량이 35% 감소한다(Ali et al., 2014; Beddow et al., 2015). 밀 붉은 곰팡이병(Fusarium ear blight)은 아시아, 북미, 유럽, 남미 등 세계 전역에서 발생하는 병으로 Fusarium graminearum (Gibberella zeae)에 의해 발생한다(Ryu et al., 1990). 붉은곰팡이병에 감염된 밀의 이삭 일부 또는 전체가 갈색으로 변하게 되고, 홍색의 분생포자층이 생기게 된다. 1988년과 2000년 사이에 미국 중서부 지역에서 밀이 붉은곰팡이병에 감염되어서, 2.7억 달러의 경제손실이 발생했다(McMullen et al., 1997; Nganje et al., 2004). 우리나라에서도 1963년 남부지방에서 발생한 대유행으로 인해 맥류 수확량의 40~80%가 감소하였고(Chung, 1975), 이후 10년주기로 발생하는 것으로 알려져 있다(Park et al., 2012). 밀 흰가루병(Wheat powdery mildew) 역시 서늘하고 습한 온대지역에서 발생하고 병원균은 Erysiphe graminis de Candolle로 알려져 있다. 밀 흰가루병은 표면에 백색 반점이 생기며 흰 가루를 뿌린 듯한 외관을 보이고, 병반이 회색과 담갈색으로 변하게 되며, 매년 전 세계적으로 10억달러 이상의 경제적 손실이 발생했다고 보고되었다(Menardo et al., 2017).
비생물학적 스트레스(Abiotic stress) 또한 밀의 생육과 수확량에 피해를 입힌다. 염, 가뭄, 온도 등의 비생물학적 스트레스로 인해 세계 농업에 커다란 영향을 주고 있고, 주요식량작물의 평균 수확률을 50% 이상 감소시키고 있다(Acquaah, 2007). 전 세계적으로 경작지의 20% 이상이 고염 스트레스의 영향을 받고, 기후 변화와 인위적 활동으로 인해 영향 받는 지역이 나날이 증가하고 있다. 현재 경작되는 밀의 대부분은 고염 스트레스에 대한 내성을 가지고 있지만, 간척지와 같은 높은 염분 수준의 지역에서는 밀 생산이 어렵다. 고염 스트레스는 종자 발아, 식물 성장, 광합성, 수분 관계, 영양소 흡수에 영향을 끼쳐서, 밀의 생육과 수량에 악영향을 준다. Chinnusamy et al. (2005)은 밀의 염분 임계수준이 6 dS m-1이며, 밀 수확량은 dS m-1 단위 증가 당 7.1% 감소할 수 있다고 보고하였다. 가뭄 또한 밀의 삼투 스트레스(Osmotic stress)를 유발하여 큰 피해를 주는 요인이며, 미국에서만 최대 80억 달러에 달하는 피해가 매년 발생한다(Dai, 2011; Fontaine et al., 2014). 밀은 고온 스트레스에도 매우 민감하여 수확량이 감소된다고 보고되어 있다(Gupta et al., 2013). 연구에 따르면 온도가 1°C 증가 할 때마다 전 세계 밀 생산량이 6% 감소한다(Asseng et al., 2014). 온도변화는 밀의 생장에 큰 영향을 미쳐서 일정 온도 범위를 넘어선 환경에서는 결과적으로 수확량의 손실로 이어지게 된다(Porter & Gawith, 1999; Thakur et al., 2010; Farooq et al., 2011). 또 다른 문제점으로 수발아 현상이 있다. 수발아는 등숙 기간에 장시간 비를 맞고 낮은 온도로 수확기 직전에 밀 낱알에서 비정상적으로 발아하는 현상이다(Groos et al., 2002). 수발아 현상이 발생한 밀 낱알에서는 외관상 과피가 파괴되고, 원맥의 밀도, 천립중이 줄어들며 병원균, 세균 번식으로 인해 밀가루의 변색이 일어난다(Martin et al., 1998). 또한 수발아 현상 때 발생한 α-amylase에 의해서 전분이 분해되어 밀가루 또는 밀 가공제품의 품질이 현저히 떨어진다(Lunn et al., 2001). 우리나라와 밀 재배환경이 비슷한 미국 북서부 지역은 등숙기간 강우량에 따라 수발아율이 16%에서 최대 69%까지 나타나는 것으로 보고되어 있다(Grunberg et al., 2002). 최근 극심한 기후 변화와 등숙기 때의 잦은 강우로 인해 국내 밀 재배에서도 수발아 위험성이 높다(Shin et al., 2013).
마지막 문제점으로 밀 저장 단백질에 의해 유발되는 밀 관련 질환(Wheat related diseases)이 있다. 글루텐 단백질은 밀 저장 단백질의 85% 이상을 차지하며 성질에 따라 글루테닌과 글리아딘으로 나뉘고, 물과 결합하여 밀 반죽의 점탄성 특성을 부여한다(Khatkar et al., 2002; Wieser, 2007). 그러나 동시에 인간의 여러 건강상의 문제를 야기하는 원인물질이기도 하다(Battais et al., 2005). 밀 관련 질환은 면역학적 발병메커니즘에 따라 자가면역질환, 알러지 질환, 비셀리악글루텐민감성(Non-coeliac gluten sensitivity)으로 나눌 수 있다(Scerf et al., 2016). 자가면역질환인 ‘셀리악병(Coeliac disease)’은 소화과정에서 소장에 잔류하는 글루텐 단백질의 항원결정기(Epitope)에 의해 면역시스템이 소장의 융모를 항원으로 인식 및 공격하게 된다. 이로 인해 소장 융모가 위축되고 평편하게 변해 두통, 설사 등 여러 증상을 보이는 병이다(Caio et al., 2019). 셀리악병의 항원인 α-글리아딘의 T세포 자극 항원 결정기는 PFPQPQLPY, PQPQLPYPQ 외 3개(Sollid et al., 2012, Tye-Din et al., 2010), γ-글리아딘은 PQQSFPEQQ, IQPEQPAQL 외 9개(Sollid et al., 2012), 그리고 ω1, 2-글리아딘은 PFPQPQQPF, PQPQQPFPW를 갖는다(Tye-Din et al., 2010). 밀 알러지 중 가장 대표적인 질환으로 밀 의존성 운동 유발성 과민증(Wheat-dependent, exercise-induced anaphylaxis, 이하 WDEIA)이 있다. 일반적인 식품 알러지처럼 먹은 즉시 증상이 나타나지 않지만, WDEIA은 음식 특이적 과민성으로서 밀로 만들어진 음식을 먹고 운동을 했을 때, 호흡곤란, 두통, 쇼크 등의 증상이 일어나는 질환이다. 주요 원인은 밀의 ω5-글리아딘과 고분자 글루테닌으로 알려져 있다(Altenbach et al., 2018). 마지막으로 비셀리악 글루텐 민감성(Non-coeliac gluten sensitivity, 이하 NCGS)의 발병기작은 아직 잘 알려져 있지 않지만, 선천적 면역 체계의 활성화와 글루텐 및 다른 밀 성분의 직접적인 세포 독성 효과가 연루되어 있다(Fasano et al., 2015; Elli et al., 2015; Leonard et al., 2017). NCGS은 밀 섭취 후 소화기계 질환, 두통, 피로, 두드러기 등의 증상을 보이는 것으로 셀리악병과 WDEIA기작 이외의 원인으로 기인되는 질환이다. 미국의 경우 밀 관련 질환을 앓는 인구가 미국 전체의 6%에 달하며 이들 질환의 치료법이 없기 때문에 이를 대체할 글루텐-프리 식품에 대한 시장성은 매년 커져서 2019년 기준으로 전 세계 76억 달러에 달한다(Igbinedion et al., 2017; http://www.statista.com; 2020년 9월 1일 기준).
위와 같이 많은 문제점에도 불구하고 여전히 밀에 대한 분자생물학적, 생명공학적 연구는 벼와 옥수수에 비해서 많이 부족한 상황이다. 옥수수의 경우 제초제 저항성 옥수수 등 생명공학 기술이 가장 많이 적용된 작물이고 벼는 중요 모델작물로써 일찍이 유전체 연구가 활발히 진행 되었지만(Ishida et al., 2015) 밀의 방대하고 복잡한 염색체의 특징 때문에 밀은 기초 유전정보를 연구하고 유전자변형 식물을 얻는데 어려움이 있다. 현재, 가장 많이 재배되고 있는 보통밀(빵밀, Triticum aestivum)은 이질6배체(Allohexaploid, AABBDD, 2n = 6x = 42)이며, 이질4배체 재배종인 T. turgidum (AABB, 2n = 4x = 28)와 야생종 A. tauschii (DD, 2n = 2x = 14)가 교배되어 생겨난 품종으로써 그 구조가 매우 복잡하고 발현되는 유전자들 간의 상호보완적 관계에 있다. 또한 밀의 유전체 크기는 약 16 Gb (1.6 X 1010)인데 이는 인간 게놈의 5배, 벼 게놈의 35배에 달하여 매우 큰 편에 속한다(Zimin et al., 2017).
이러한 복잡성과 방대성을 가진 밀 유전체는 차세대염기서열분석법(Next generation sequencing 이하 NGS)을 통해서 분석이 이루어졌고, 이후 2017년과 2018년에는 16 Gb에 달하는 밀 게놈의 분석이 완료되어 유전체 연구의 새로운 장이 열리게 되었다(Zimin et al., 2017; IWGSC, 2018). 새로운 유전자 정보를 얻을 수 있게 되었고 각 유전자 기능에 대한 연구 역시 RNA간섭, 과발현체 제작, CRISPR/Cas9 system을 비롯한 다양한 유전자편집기술 중심으로 활발하게 진행되고 있다. 특히 유전자편집기술을 적용하기 위해 외래 유전자의 도입에 많은 영향을 주고 있다. 이에 필요한 다양한 형질전환방법들이 고안되었고, 특히 아그로박테리움 형질전환법과 유전자총 형질전환법이 대표적이다.
본 총설에서는 밀 생명공학 연구에 많이 사용되는 형질전환법인 아그로박테리움 형질전환법(Agrobacterium-mediated wheat transformations)과 유전자총 형질전환법(Biolistic wheat transformation)에 관한 전반적인 연구방법 및 이를 응용한 최신 연구결과에 관해 기술할 예정이다.
식물형질전환(Plant transformation)
외부의 DNA (또는 유전자)를 식물체나 세포 수준에 삽입하여 식물의 유전 형질을 바꾸는 것을 형질전환이라고 한다. 전통육종방식을 통해 품종개량을 해오던 인류는 1980년대를 지나면서 육종 분야에 커다란 변화를 맞이하게 된다. 토양 미생물 중 자연 상태에서 식물세포를 감염시키는 아그로박테리움을 발견하게 되고, 이 미생물이 제한효소를 통해 유전자를 재조합하는 것을 알게 되었다(Gelvin, 2003). 이러한 발견과 더불어서 유전자재조합기술의 발전이 더해져서 새로운 유전자를 도입한 형질전환 식물체(transgenic plant)를 만들 수 있게 되었다. NGS 기술의 발달로 인해 새로운 유용 유전자 정보를 쉽게 얻게 되었고, 이들 유전자에 대한 기능을 밝혀 새로운 생명공학 품종을 개발하는데 식물형질전환 기술은 필수 불가결한 요소이다.
식물형질전환법에는 아그로박테리움법, 유전자총 이외에도 전기충격이나 폴리에틸렌 글리콜(Polyethylene glycol, 이하 PEG)를 이용한 일과성발현법(Transient expression trnasformation), 미세 주입기를 이용한 직접주입법 등의 방법들이 있다(Bates, 1995; Jackson et al., 2003).
아그로박테리움 밀 형질전환(Agrobacterium-mediated wheat transformations)
아그로박테리움(Agrobacterium tumefaciens)은 작물 뿌리와 줄기의 경계부에 근두암종병(crown gall disease)을 일으키는 원인균이다. 아그로박테리움은 그람음성균이며 운동성이 있는 토양세균으로 식물뿌리에서 분비되는 영양분에 의존하여 살아가는데 영양분이 부족해지면 식물의 상처 난 부위로 직접 침투하여 기생하는 특징을 가지고 있다. 식물체에는 감염된 이후 줄기나 뿌리에 크라운 골(crown gall)이라는 혹이 생기게 된다. 근두암종병은 지난 1세기동안 원예작물에 큰 골칫거리였는데 체리, 사과, 포도와 같은 다년생 원예작물의 수확량에 커다란 타격을 입혔기 때문이다(Kennedy, 1980; Lopatin, 1939; Ricker et al., 1959; Schroth et al., 1988). 아그로박테리움 형질전환법은 토양 내에 존재하는 미생물인 Agrobacterium tumefaciens의 Tumour inducing (Ti) 플라스미드 유전자를 도입에 이용하는 방법이다. Ti플라스미드 중에 크라운 골을 만드는 T-DNA 영역에 유용 유전자를 재조합하여 아그로박테리움에 도입하고, 도입된 아그로박테리움을 식물에 감염시켜서 유용 유전자를 식물 염색체에 도입하여 형질전환 식물체를 만들었다(Van Larebeke et al., 1974; Zaenen et al., 1974; Chilton et al., 1977, 1978; Depicker et al., 1978). 외래 유전자인 유용 유전자가 도입된 형질전환 식물체는 항생제를 이용하여 조직배양 방법을 통해 선발과정과 재분화 과정을 거친 다음 완전한 형질전환 식물체가 된다(Fig. 1).
최근에는 아그로박테리움에 의한 유전자 도입 효율을 높이기 위해 감염성이 높은 Agrobacterium tumefaciens 균주를 사용하고, 운반체 구조를 변경하는 등 다양한 시도가 이어지고 있다.
아그로박테리움 형질전환법은 주로 쌍자엽 식물에 이용되고, 벼를 비롯한 단자엽 식물의 형질전환에도 이용되고 있다. 1983년 최초로 아그로박테리움 형질전환법을 이용하여 항생제 카나마이신 저항성 페튜니아의 개발에 성공하였다(Fraley et al., 1983). 그 후 Cheng et al. (1997)이 밀을 대상으로 한 아그로박테리움 형질전환에 최초로 성공하였다. 곡류는 아그로박테리움 균의 자연숙주가 아니기 때문에 그에 맞는 벡터를 제작하고 꾸준히 수정해왔다(Cheng et al., 2004).
본 총설에서 기술할 밀 아그로박테리움 형질전환은 Hayta et al. (2019)의 방법을 토대로 작성하였다. 먼저, 개화 후 14일쯤 된 미성숙 낱알을 채취한 후 포영, 내영을 제거한다. 그 후 70% 에탄올로 1분, 1% 표백제로 10분간 소독한 후 멸균수로 3번 씻어낸다. 이후 미성숙 낱알로부터 미성숙 배를 적출한다. 적출한 미성숙 배는 2.0 ml 튜브로 옮긴 후 액체 MS 배지를 담는다. 그 후 14,000 rpm, 4°C에서 10분간 원심분리해준다. 배지를 제거 한 후에 미리 배양해 둔 유용유전자가 삽입된 아그로박테리움을 튜브에 담아준다. 튜브를 30초간 위아래로 뒤집어서 충분히 섞이도록 한 후 20분간 상온에서 배양한다. 현탄액을 페트리 접시에 붓고 나서 용액을 피펫으로 제거한 후 미성숙 배를 준비된 공동배양배지에 옮겨서 암조건, 24°C에서 3일간 배양한다. 이때 미성숙 배의 배반(scutellum)이 위로 올라오도록 옮겨야 한다. 이후 배축을 절제한 후 2,4-dichlorophenoxyacetic acid (이하 2,4-D)가 첨가된 캘러스 유도 배지로 옮겨준 후 암조건, 24°C에서 5일간 배양한다. 이후 형질전환 식물체 선발을 위한 15 mg/L hygromycin이 첨가된 새 캘러스 유도 배지로 미성숙배를 옮기고 암조건, 24°C에서 2주간 배양한다. 그 후 30 mg/L hygromycin이 첨가된 캘러스 유도 배지로 옮긴 후 암조건, 24°C에서 3주간 배양한다. 이후 재분화 배지로 옮긴 후 광조건, 24°C에서 배양한다. 재분화 된 새싹과 뿌리가 보이면 뿌리 유도 배지로 옮겨 광조건, 24°C에서 배양하고, 뿌리가 유도된 형질전환 식물체를 흙으로 옮겨 생장하게 한다.
아그로박테리움법으로 도입된 유전자의 고정이 용이하고 유전자침묵현상(gene-silencing)도 적은 편이다. 하지만 비숙주 식물인 단자엽 식물에서는 그 효율이 떨어지며 품종, 계통에 따라 재분화 효율, 형질전환 효율이 달라진다. 그리고 배양기간에 따른 변이체 발생, 형질전환 식물체 획득까지 소요되는 시간이 긴 단점도 있다(Hiei et al., 1994; 1997; Hirochika et al., 1996; Rachmawati & Anzai, 2006).
유전자총(Biolistic wheat transformation)
유전자총은 다른 개체를 이용하지 않고 유전자를 직접 도입하는데 가장 효율적으로 쓰이는 형질전환 방법이다. 1987년에 양파를 대상으로 처음 실험에 성공하였고 대두(大豆)와 담배를 통해 첫 형질전환 식물체를 얻었으며 이후 화본과 작물의 형질전환을 만드는데 많이 사용되었다(Sanford et al., 1987, 1988; Christou et al., 1988; Klein et al., 1988). 이후 유전자총은 식물 뿐만 아니라 박테리아, 곰팡이, 동물 세포를 비롯하여 엽록체, 미토콘드리아와 같은 세포소기관까지 다양한 재료에도 사용 된다(Smith et al., 1992; Toffaletti et al., 1993; Johnston et al., 1988, 1991; Boynton et al., 1988).
밀을 대상으로 유전자총을 실시한 첫 사례로 미국 플로리다 대학교에서 수행한 실험이 보고되어 있다(Vasil et al., 1992). 밀의 형질전환을 위해 식물체의 여러 부위들이 사용되었으며, 현재 유전자총을 적용하는 보편적인 목표 부위는 종자의 배(embryo)이며 이를 이용한 배발생 세포배양(embryogenic cell culture)는 안정적으로 형질전환 된 식물체를 얻을 수 있는 효과적인 방법 중 하나로 쓰여지고 있다(Vasil et al., 1992).
유전자총 기기 중에 보편적으로 Bid-Rad사의 “PDS1000/ He”를 가장 많이 사용한다(Sanford et al., 1991). 본 총설에서 기술한 유전자총 형질전환법은 미국 농무성의 Altenbach & Allen (2011)의 방법을 토대로 기술 하였다. 밀 개화 후 12~14일 된 미성숙 종자를 채취한 후 포영, 내영을 제거한다. 70% 에탄올에 Tween-20을 첨가하고 1분동안 음파처리 한다. 70% 에탄올에서 15분, 20% 표백제로 10분간 소독한 후 멸균수로 3번 세척하였다. 소독한 미성숙 종자에서 미성숙 배아를 적출한다. 적출한 미성숙 배아의 안정화를 위해 1 mg/L 2,4-D가 첨가된 MS 배지에서 4~6일간 암처리하였다. 유전자총 형질전환법을 실시하기 4시간 전에 0.5 M sucrose가 첨가된 Bombardment 배지로 옮겨준다. 그 후 직경이 0.6 μm gold particle을 대량의 유용 DNA를 코팅한다. gold particle와 유용 DNA를 코팅할 때 에탄올이나 염화칼슘(CaCl2)을 이용하고, 코팅된 유용 DNA및 gold particle 구조 변형을 막기 위해 스퍼미딘(spermidine)을 첨가해 준다. 1100 psi헬륨가스 압력으로 macrocarrier에 도말, 코팅된 유용DNA는 미성숙 배아에 고속으로 도입된다. 형질전환시킨 미성숙 배아들은 암조건에서 24시간 후 처리 후, 캘러스 유기를 위하여 1 mg/L 2,4-D가 첨가된 MS 배지로 옮겨 암조건으로 2주 간격으로 계대배양하여 총 4주 동안 캘러스를 유기한다. 캘러스에서 재분화를 위해 2,4-D (0.1 mg/L)와 6-Benzylaminopurine (이하 6-BA, 0.1 ug/L)가 첨가된 MS배지로 유기된 캘러스를 옮긴 후 광조건에서 2주 간격으로 총 8주동안 계대배양하여 캘러스에서 녹점과 잎을 유도한다. 캘러스에서 재분화된 식물체들은 1/2 MS 배지로 옮겨져 뿌리 생장을 촉진시키고, 흙으로 옮겨져 온실에서 성체로 자라게 된다(Fig. 2).
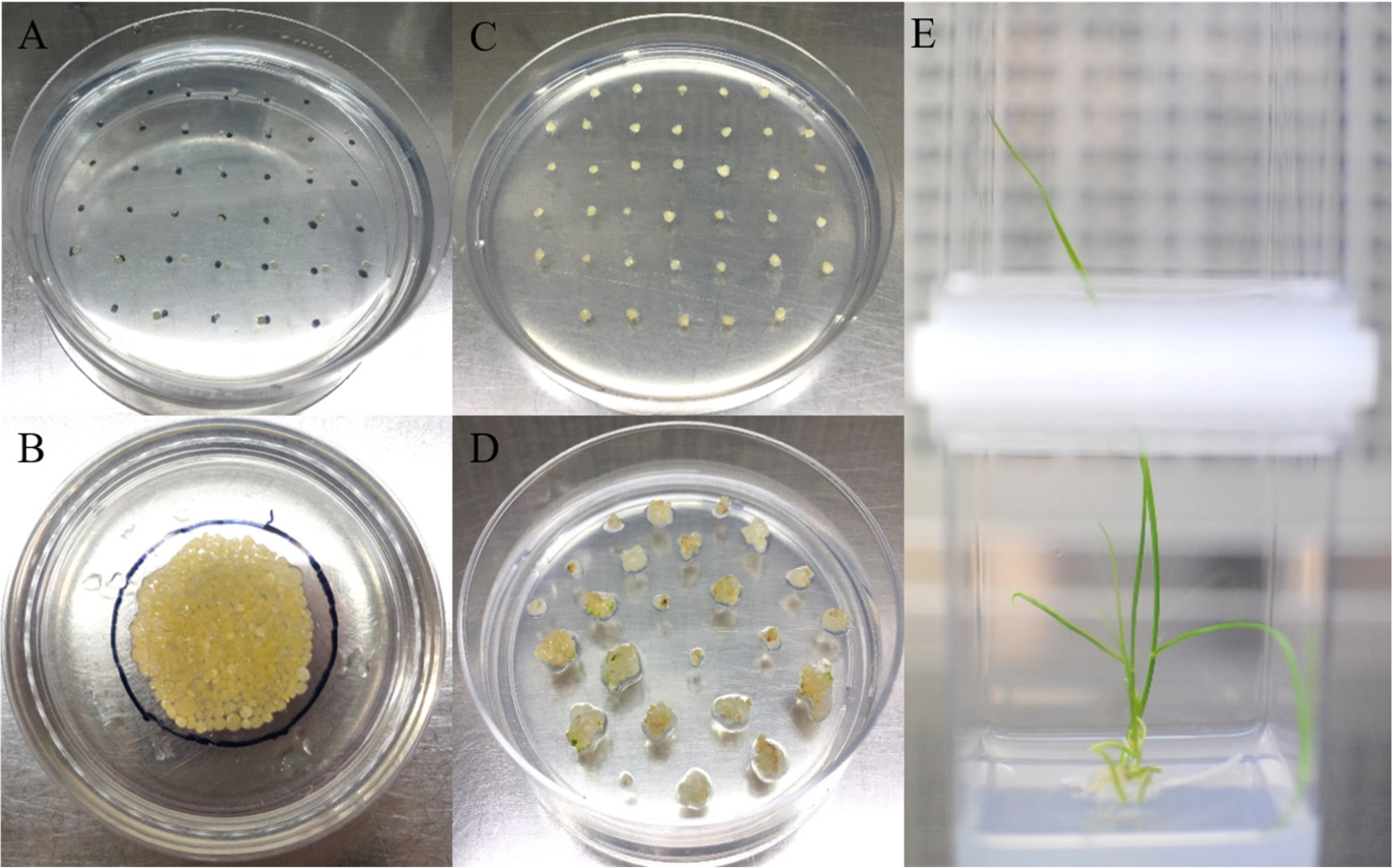
Fig. 2
Procedure for biolistic wheat transformation. (A) Excised immature embryos being cultured on Murashige and Skoog (MS) media. (B) Explants collected on sucrose media before being treated using biolistic transformation. (C) Explants turned into calli on MS media. (D) Regenerated calli showing green spots which will turn into shoots. (E) Plantlets grown on MS media.
유전자총 형질전환법의 장점은 아그로박테리움 형질전환법과 달리 목적유전자를 특수한 벡터 유전자에 클로닝 할 필요없이 빠르게 진행 할 수 있다. 세포나 조직에 영향을 최소화 할 수 있고 목적 부위가 핵 뿐만 아니라 엽록체 등 다양한 곳에 삽입이 가능하다. 하지만 고가의 기기가 필요하며 각 밀 품종 마다 재분화조건에 따른 호르몬의 농도 등 조직배양조건을 달리해야 한다. 하지만, multi-copy integration의 단점도 있다(Christou, 1997; Makarevitch et al., 2003).
밀 형질전환을 이용한 밀 생명공학 연구현황
1980년대 아그로박테리움과 유전자총 형질전환법 개발되었다. 이 기술들은 NGS를 통한 유전자 정보들을 얻게 되면서 식물병, 비생물학적 스트레스. 밀 관련 질환을 극복하기위한 연구에 적극적으로 활용되었다. 아그로박테리움 형질전환법을 활용한 연구사례들은 Table 1, 유전자총 형질전환법을 활용한 연구사례들은 Table 2에 정리하였다.
Table 1.
Recent studies of wheat transformation using Agrobacterium-mediated transformation.
Cultivar | Type of expression | Target gene | Description | Reference | |
Plant diseases |
CYR31, CYR23, Su11 | Overexpression |
ATG8 (Autophage- related 8) |
Confirmation of the function of increasing resistance to rust. | Mamun et al., 2018 |
Abiotic stress | Sohag 2 | Overexpression | P5CS |
Proline-induced osmotic inhibitory increase in transgenic lines, resulting in increased resistance to salinity | Sawahel & Hassan, 2002 |
Hesheng 3 | Overexpression |
NHX1 (Vacuolar Na+/H+ antiporter) |
Improved grain yield in soil with high salt conditions, reduced Na+ levels in leaves, and improved salt resistance | Xue et al., 2004 | |
Fielder | Overexpression | WRKY2 |
Increased expression of drought-related genes (DREBs, GST6, ERF5a, TIP2, WRKY19), resulting in increasd drought tolerance | Gao et al., 2018 | |
JN177, SR3 | Overexpression, RNAi-mediated silencing | CYP81D5 |
Elimination of ROS by cytochrome P450’s CYP81D5, resulting in improved resistance to high salts | Wang et al., 2020 | |
Fielder | CRISPR/Cas9 | Qsd1 |
Confirmation of increase in seed dormancy retention in transgenic lines | Abe et al., 2019 | |
Wheat-related disease | Fielder | CRISPR/Cas9 | α-, γ-gliadins |
Confirmation of decreased contents of each α-γ-gliadin and ω-gliadin | Jouanin et al., 2019 |
Table 1.
Recent studies of wheat transformation using Agrobacterium-mediated transformation.
먼저, 아그로박테리움 형질전환법을 활용한 연구는 다음과 같다. 식물병 연구에서는, 밀의 자가포식(Autophagy)이 식물병저항성과 관련 있으며 이를 조절하는 Autophagy-related 8 (ATG8) 과발현을 유도한 형질전환 식물체를 제작하였다(Mamun et al., 2017).
비생물학적 스트레스 연구에서는, 프롤린 생합성을 조절하는 pyrroline-5-carboxylate synthetase (P5CS)을 과발현 했을때, 프롤린이 삼투보호제로써 작용하고 염 스트레스에 저항성이 증가하였다(Sawahel & Hassan, 2002). 또한, 애기장대에서 액포 Na+/H+역수송체 발현을 조절하여 액포의 Na+을 조절하는 NHX1의 유전자를 밀에서 과발현식물체를 제작한 결과, 고염 조건의 토양에서 밀 수확량이 증가하였고, NHX1과발현식물체의 잎에서 Na+함량 수준이 감소하여 내염성이 향상되었다(Xue et al., 2004). WRKY 전사인자들은 비생물학적 스트레스와 식물의 생장에도 영향을 준다고 알려져 있다(Jiang et al., 2012; Zhu et al., 2013; Tripathi et al., 2014; Xu et al., 2016). 밀의 WRKY2 전사인자의 과발현식물체에서 가뭄 스트레스 관련 유전자들(DREBS, GST6, ERF5a, TIP2, WRKY19)의 발현이 증가하여 가뭄 스트레스 저항성 증가하였다(Gao et al., 2018). 또한, 지구온난화로 인한 기후변화는 밀의 수발아 현상을 야기시키고 있는데 CRISPR/Cas9시스템 이용하여 종자의 휴면을 조절하는 TaQsd1유전자를 밀에서 형질전환체를 제작하였다. 8개의 돌연변이가 일어난 형질전환 식물체와 3개의 다중 돌연변이가 일어난 형질전환 식물체를 얻을 수 있었고 50% 종자발아에 걸리는 시간이 일반 밀보다 5일 이상 지연된 것으로 보고되었다(Abe et al., 2019). 밀 관련 질환 연구에서는 셀리악병의 항원결정기가 되는 α, γ-글리아딘에 대한 목적 유전자를 CRISPR/Cas9시스템을 이용하여 형질전환 식물체를 제작하였다. 그 결과, 3.3% 효율로 α, γ-글리아딘 발현이 저감 된 형질전환 식물체를 얻었다(Jouanin et al., 2019).
유전자총 형질전환법을 활용한 연구동향을 보면 식물병 연구에서는, β-1,3-glucanase transgenes유전자가 과발현하는 식물체에서 방어기작 유전자가 과발현 시킴으로써 붉은곰팡이병에 대한 저항성이 증가하였다(Mackintosh et al., 2007). 애기장대에서는 Enhanced disease resistance1 (EDR1) 유전자가 흰가루병 저항성을 방해하는 것으로 보고되어 있다(Frye et al., 2001). 밀의 EDR1을 타켓 유전자로 하여CRISPR/Cas9 시스템을 통해 EDR1돌연변이를 유도하여 5개의 흰가루병 저항성을 보이는 형질전환 식물체를 제작했다(Zhang et al., 2017).
비생물학적 스트레스에서 유전자총 형질전환을 활용한 연구 사례를 보면, 글리신 베타인(Glycine betaine)을 조절하는 betaine aldehyde dehydrogenase (BADH) 유전자를 과발현 시켰다. 글리신 베타인의 과발현 형질전환 식물체는 광합성에 영향을 주는 가뭄, 온도 스트레스에 대한 내성이 증가했다(Wang et al., 2010). 또한, 식물 생장에서 철분은 매우 중요하므로 철분 함량조절 단백질인 ferritin발현을 조절하는 FER-5B 유전자를 연구하였다. FER-5B 과발현식물체에서는 Ferritin단백질의 증가로 활성산소가 제거되어 열 스트레스에 대한 내성이 증가했다(Zang et al., 2017). 또한, 밀의 수확량과 품질에 영향을 주는 유전자에 대한 연구도 이루어 졌다. DENSE AND ERECT PANICLE 1 (DEP1) 는 밀 수확량, 질소 흡수 및 스트레스 내성 조절을 관여한다고 보고되었다(Xu et al., 2016). 전사인자 NAC2는 가뭄과 염 스트레스를 조절기능이 있다고 보고 되어 있다(Mao et al., 2012). TaDEP1, TaNAC2를 목적 유전자로 CRISPR/Cas9 시스템을 통해 형질전환 식물체를 제작하였고, 각각 2% 효율로 돌연변이가 유도된 형질전환 식물체를 제작했다. 또한 TaDEP1 돌연변이 식물체에서 평균 36.7 cm로 일반 밀의 65% 크기로 생장했다(Zhang et al., 2016).
CRISPR/Cas9시스템을 이용하여 글루텐 알러지 병 중 하나인 셀리악병을 유발하는 α-글리아딘의 함량을 32%에서 최대 82%까지 감소한 형질전환 식물체를 제작하였다(Sánchez- León et al., 2018). 밀 의존성 운동 유발성 아나필락시스(WDEIA)를 유발하는 ω-5 글리아딘 유전자를 RNA간섭(RNA interference; RNAi) 시스템을 이용하여 54개의 ω-5 글리아딘 함량이 감소한 형질전환 식물체를 얻을 수 있었다(Altenbach & Allen, 2011). α-amylase/trypsin inhibitors (ATI)는 제빵사의 천식 및 NCGS에 관여하다고 알려져 있다. RNA간섭을 통하여 α-amylase/trypsin inhibitors (ATI) 유전자의 발현이 감소하는 형질전환체를 제작하였고, ATI 발현량이 일반 밀보다 80% 감소한것으로 보고되었다(Kalunke et al., 2020).
16 Gb에 달하는 밀 게놈의 분석이 차세대염기서열분석법을 통해서 분석이 완료되어 유전체 연구의 새로운 장이 열리게 되었다(Zimin et al., 2017; IWGSC, 2018). 최근 식물병, 비생물학적 스트레스(Abiotic stress), 밀 관련 질환 등 극복해야 할 여러가지 문제점에 대한 연구가 RNA간섭, 과발현체 제작, CRISPR/Cas9 system을 비롯한 다양한 식물생명공학기술을 통하여 활발하게 진행되고 있다. 전통육종법과 더불어 발전된 식물생명공학 기술을 통해 이러한 어려움을 극복해 나갈 수 있을 것으로 기대한다.
적 요
밀은 세계 3대 작물 중 하나이고, 전 세계 인구가 소비하는 영양 칼로리의 20%를 담당하는 등 중요한 식량작물이지만 이질 6배체의 복잡한 염색체와 약 16 Gb의 방대한 유전체 크기로 인해 다른 작물들에 비해 분자생물학 및 생명공학연구가 많이 부족한 상황이다. 최근 최신의 차세대염기서열분석법에 의한 밀 유전체 분석이 이루어져 유용한 유전자를 쉽게 얻을 수 있게 되어 여러 방면에서 밀 생명공학연구가 가속화 되고 있다. 본 리뷰에서는 밀의 유전자의 기능을 밝혀 새로운 기능의 생명공학 밀을 육성하는데 필수 불가결한 기술인 밀 형질전환에 대해 상세히 기술하였다. 또한 밀 생명공학기술과 형질전환에 의해 전통육종에 의해 해결하기 어려운 식물병, 비생물학적 스트레스 및 밀 관련 질환을 극복하기 위한 최신의 연구 결과에 대해 서술하였다.